Saving Dr.
Schrödinger's Cat
by
Danny
J. Krebs
Quantum theory began in the early
1900s as physicists looked at how
atoms interact with light. It is
called quantum theory because light
energy was found to only be adsorbed
or emitted in discrete amounts, called
quanta, the plural of the Latin quantum,
from quantus, "how
much". It seemed natural to
think that there was a light particle
called the photon that carries a fixed
amount of energy, the amount of which
depends on the color of light involved
(Gribbin 82-85). By invoking a
particle model, Einstein, Planck, and
others were able to explain much about
the interactions of light with
matter. On the other hand, it
was known that light also exhibits
wave behavior, similar to the behavior
of waves on the surface of a
lake. Light appears to
exist as point-like particles when
interacting with matter, but to exist
as waves when it travels from place to
place. This is called the
wave-particle duality. The conversion
of the wave to particle is sometimes
called a "wave function
collapse." I will use the terms
"wave function collapse" and
"wave-particle duality"
interchangeably, although they imply
somewhat different mental
pictures. Electrons, which were
once thought to exist only as
particles, were also found to have
wavelike properties. Wave-particle
duality appears to be a characteristic
of all forms of matter and
radiation. The application of
wave equations to matter, especially
electrons, accounts for much of the
success of 20th century physics,
giving us useful devices like
transistors, computers, and lasers
(Ghirardi 12-15).
In
the early decades of the twentieth
century, the physics community was
divided regarding the proper view of
the wave-particle duality and the
statistical nature of quantum
theory. Lively debates occurred
in the 1930s, pitting Albert Einstein
against the Danish physicist Niels
Bohr. Einstein could not accept
quantum theory as being complete
(Kaiser 3-13; Herbert 200). He
is famous for saying that "God does
not play with dice" (Hermanns 58), but
that quotation does not do justice to
the depth and complexity of his
misgivings. Bohr believed that
quantum theory was complete as it
stood and that no tinkering with its
basic framework was needed. (1)
Figure 1
The
crux of the disagreement between
Einstein and Bohr can be understood by
looking at what happens as light
passes through a pair of narrow,
closely spaced slits (see Figure
1). Provided that the light is a
single color, a wavy intensity pattern
is seen on a screen placed at some
distance from the slits. There
is a central peak exactly equidistant
from the two slits; there are valleys
on each side; and somewhat smaller
peaks repeating at fixed
intervals. The peaks in
the pattern are points at which the
waves from each slit arrive with their
positive crests overlapping. The
valleys correspond to the wave crests
from one slit arriving overlapping
negative troughs from the other slit.
If either slit is blocked, the wave
pattern disappears immediately.
Here
is the problem. We can reduce the
light intensity so that we see
point-like flashes on the screen
indicating the arrival of individual
photons, shown as dots in Figure
1. If we add up the arrival of
photons over a long time, we still see
the same peak/valley intensity
pattern. How did a single light
particle, which presumably travelled
through only one of the two slits
"know" that it was not supposed the
land in a valley? How did it
"know" that the other slit was open
and not blocked? Did the photon
somehow go through both slits?
Bohr's answer was that it makes no
sense to talk about a point-like
particle having a life in the setup
before it is detected on the
screen. Between the source and
the screen, light is a wave. The
presence of the screen causes the
particle to materialize at specific
points and the distribution of those
points is governed by a "wave
function" easily calculated from the
width and separation of the two
slits. Furthermore, Bohr
asserted that the interference pattern
is due to the experimenter's ignorance
of the photon path. Any attempt
to detect which path the photon takes
would instantly cause the wavy pattern
to disappear. In other words,
the very act of observation affects
the distribution of detection
events. Einstein did not buy
it. To him, light particles are
real, point-like entities that must
take certain trajectories through the
system.
It
was generally assumed that Bohr had
won all the arguments and that
Einstein was just being difficult by
not embracing Bohr's point of view,
which is called the Copenhagen
Interpretation. Quantum theory
was too successful to worry about the
best way to view the wave-particle
duality. Quantum calculations
explain everything from the structure
of atoms to the properties of
semiconductors to the numerical values
of certain constants of
nature. From the 1940's
into the 1970's, the vast majority of
physicists believed that Bohr's view
of the wave-particle duality must be
the correct one. Questioning the
Copenhagen interpretation was
considered to indicate a deficit in
one's education, intellect, or
both. Additionally, in the
1940's one of the greatest
mathematicians of the twentieth
century, John Von Neumann, developed a
"proof" that sentient (i.e. human)
observation was necessary to collapse
the wave function. Von Neumann's
proof had a logical error, but due to
the prestige of its author, the error
was not discovered for several years
(Ghirardi 197-202). In recent years,
mainstream scientific thought has
evolved from a knee-jerk acceptance of
the Copenhagen Interpretation to a
more balanced view. This evolution is
in no small part due to the work of a
few courageous researchers persevering
against the orthodox view formed in
the 1930s.
Other
Models and Bell's Theorem
In
the 1950s, David Bohm, a
well-respected physicist, produced a
model that duplicates the results of
quantum theory but allows particles to
have real trajectories. Just as
in orthodox quantum theory, Bohm's
model assumes a quantum wave function,
but rather than dictating
probabilities for photon arrival at
the screen, the wave function creates
a force that pushes real particles
through the setup. In Bohm's
model, the apparent uncertainty of
quantum mechanics comes from
uncertainty in the initial position of
the particle. Bohm's model
generated a certain amount of
interest, but it was untestable
because its predictions exactly match
those of the Copenhagen model.
Bohm was involved in the Manhattan
Project, but his earlier membership in
Communist organizations became an
issue during the McCarthy era.
Bohm was tried and acquitted for his
refusal to answer questions before
Congress. He later emigrated to
Brazil, then to Israel, then to Great
Britain.
In
1964 an Irish physicist, John Stewart
Bell, discovered a tool for testing
one of Einstein's objections to the
Copenhagen interpretation.
Certain processes result in pairs of
particles that are guaranteed to have
correlated attributes ("attribute" is
a term physicists use for properties
associated with a particle, such as
velocity, position in space, energy,
and spin); for instance, certain
radioactive materials simultaneously
emit pairs of electrons that travel in
opposite directions with identical
speed and opposite spins. In a
1935 paper, Einstein had speculated
that measurements on pairs of such
particles could overcome measurement
limitations fundamental to quantum
mechanics, but performing such
measurements on the attributes
envisioned by Einstein would be very
difficult. Bell had the idea of using
electron spin as the attribute that
could be used to put quantum theory to
the test. Because of the complexities
of performing spin measurements on
correlated pairs of electrons, the
first tests of Bell's theory were
performed on light particles, using
polarization as the tested
attribute. Pairs of photons from
certain sources are guaranteed be
emitted at exactly the same time,
travel in exactly opposite directions,
and have identical
polarizations. These pairs are
called phase-entangled photons.
(2)
At
this point, the reader needs to know a
few things about polarized
light. Polarization indicates
the direction perpendicular to the
direction of travel that is associated
with the optical disturbance. An
analogy would be to think of the light
particles as Frisbees and polarizing
filters as picket fences that can be
oriented with the pickets tilted
horizontally, vertically or at any
angle in between. Only
vertically spinning Frisbees would
make it through a picket fence with
vertical pickets. Only
horizontally spinning Frisbees would
make it through a picket fence laid on
its side. The analogy is not
perfect. Unpolarized light
always appears to be composed of
particles with half its photons
polarized one direction and half in
the perpendicular direction,
regardless of which two orthogonal
directions are chosen. It is as
if half of all the Frisbees in the
world were spinning one way and half
the other way, regardless of how we
tilt the fence. (3)
Bell's work showed that quantum theory
predicts that if polarization
measurements are performed on both of
two phase-entangled photons, the
results of measurements made on the
polarization of one photon depend on
the type of polarization measurement
being performed on its phase entangled
partner, regardless of where or when
that other measurement is
performed. The measurements
could be performed on opposite sides
of the earth and the effect of the
polarizer settings on correlations
would still be there. There is,
however, no "agreement" between the
two particles at the time of their
separation that would permit the
correlation of the subsequent
polarization measurements to follow
the predictions of quantum
theory. Somehow information
about the type of polarization
measurement being performed on the
first photon must be transmitted to
affect the results of measurements
performed on the partner photon.
This is what Einstein called "spooky
action at a distance." This
"spooky action at a distance" violates
one of the treasured ideas of physics:
local causality. According to
local causality, no physical effect
can propagate faster than the speed of
light. It was thought that
relativity theory forbids violations
of local causality, so there appeared
to be a direct conflict between
quantum theory and relativity
theory. Bell's theory is
considered to be one of the great
achievements of twentieth century
physics, but Bell died young, just as
his work was gaining
recognition. The Nobel committee
was considering him for an award just
prior to his death, but Nobel prizes
are never awarded posthumously.
It
was eight years before John Clauser at
Berkeley carried out an experimental
test of Bell's idea. The "spooky
action at a distance" predicted by
quantum theory was indeed
present. A later
experiment performed by Alain Aspect
in France showed that non-local
correlations occur even when the
choice of polarizer angle is made
after the photons are already in
flight and no light-speed signal of
polarizer settings could pass between
the two polarizers. Einstein had
intended that experiments on
phase-entangled particles would be a
challenge to the accepted precepts of
quantum theory. Instead,
experiment has shown that quantum
theory has the power to overrule the
concept of local causality.
One
might think that Bell's discovery
could possibly be used to send
messages faster than the speed of
light, but the theorists tell us that
such schemes must fail. There is
no information content in the data
recorded on one side of the
experiment. Information about
the polarizer settings is only
contained in the correlations of the
two records, which can only be
accomplished via normal communication
channels. Nevertheless, somehow
the choice of measurement on one
photon stream affects the results of
measurements on the other photon
stream. Bell type experiments
have been performed on electrons and
even whole molecules with similar
results, so the nonlocal correlations
predicted by Bell's theorem are not
confined to photons. There are
similarities between the non-local
correlations seen with phase
entanglement and the questions raised
by the twin slit experiment. In
both cases, the quantum wave function
appears to collapse to give a definite
result only when forced to do so by a
measurement, and the wave function
collapse occurs simultaneously over
all space, even when no light speed
signal could connect regions of space
that the wave function occupies.
The
field of quantum information theory
has come into being in the last thirty
years partly due to insights regarding
quantum entanglement. Quantum
information theory has found practical
application to secure communications
and may someday lead to faster
computers.
The
experiments of Clauser and Aspect
exclude so called local hidden
variable theories, theories for which
the particles are pre-programmed to
react certain ways to certain
tests. They also illustrate the
absurdity of an observer-based theory
of wave function collapse. Can
anyone really think that Aspect's
equipment did not register the
correlation signals until Dr. Aspect
observed the results? These
experiments do not answer the
question: "When does a wave become a
particle?" or equivalently, "Where
does the wave function collapse
occur?" In order to examine
these questions, we must meet Dr.
Schrödinger's cat.
Schrödinger
's Cat
Erwin Schrödinger was one of the true
fathers of quantum
mechanics. His equation
for computing the quantum wave
function of material particles is one
of the most famous equations in
science. In the Einstein/Bohr
debates, he was firmly in Einstein's
corner. Schrödinger conceived of
a thought experiment in which his cat
was confined inside a steel box with a
radioactive sample that had a 50/50
chance of emitting a gamma ray in an
hour's time. A radioactive sensor in
the box is attached to a hammer that
will break a cyanide capsule and kill
the cat if the gamma ray is
emitted. After an hour,
the experimenter lifts the lid of the
box and discovers whether the cat is
alive or dead. (4)
Quantum theory maintains that it is
impossible, even in principle, to know
whether a radioactive nucleus will
decay in a given time frame. In Bohr's
view, during its hour in the box the
cat is in a quantum superposition of
states. It is neither alive nor
dead. Only by lifting the lid of
the box and looking inside do we put
the cat in a definite quantum state,
alive or dead.
Cat lovers will be glad to know that
no actual felines have been harmed in
attempts to resolve the issue.
Schrödinger came up with the scenario
merely as a reductio ad absurdum
argument to highlight what he saw as a
problem with Bohr's understanding of
quantum theory.
There are a number of possible
solutions to the Schrödinger cat
paradox. One is called the
"relational model". The idea in the
relational model is that when the
experimenter lifts the lid, his wave
function interacts with the cat's wave
function. There then exists a
backwards-in-time chain of wave
function collapses going back to the
radioactive nucleus.
Another alternative is the so-called
many worlds interpretation. When
the radioactive nucleus does, or does
not, decay; the universe splits into
two parallel universes, one in which
the cat is dead and one in which he is
alive. When the experimenter
lifts the lid, he finds out which
universe he is in. Science
fiction writers love that
interpretation, as do some
theorists.
Many
scientists believe that there must be
a dividing line between quantum
uncertainty and macroscopic certainty
(Penrose 225-99). According to
this view, when the microscopic
quantum event becomes observable in
the larger world, the microscopic wave
function collapses. Scientists
have looked toward something called
de-coherence theory to explain the
existence of this boundary. (5) A
group in France has modeled a
particular type of quantum measurement
with both the particle and measurement
system receiving a quantum
treatment. The system
consisting both the particle and its
measuring device briefly exists in
what is called a meta-stable state
(Nieuwenhuizen 1-166). Meta-stable
states are states of unstable
equilibrium, analogous to a pencil
balanced vertically on a flat
surface. The meta-stable state
rapidly collapses into a definite
classical result due to interactions
within the system. This result
shows it is possible for a strict
application of quantum theory to lead
to what appears to be a classical
result. The evolution of a
quantum measurement may be
deterministic, but calculating the
result may be undoable because of the
very complex nature of the
interactions.
Expert opinion varies on the
significance of de-coherence theory in
resolving the Schrödinger cat
paradox. If we observe the spin
of an electron, can we really say that
the particle had that spin? Or
can we only say that of the
statistical possibilities passed on to
the measurement apparatus by the
particle, the measurement apparatus
chose that spin? Did
causality operate in reverse, going
from the measurement apparatus back to
the particle? De-coherence
theory resembles the Copenhagen
Interpretation with the role of the
observer replaced by any large object
with which the quantum particle
interacts. There is a critical
difference, however: the "wave
function collapse" has a physical
basis apart from the observer.
The moon is still there even when no
one is looking.
Something called Wheeler-Feynman
Absorber Theory might provide clue
about how to deal with the
retro-causality issues. In this
theory each photon absorption-emission
event occurs due to a "handshake"
between the emitting atom and the
absorbing atom. Waves traveling
forwards and backwards in time
interfere with one another in such a
way as to mimic the wave behavior seen
in conventional models. Quantum
theories built around these ideas are
called Transactional Models (Gribbin
237-247), or Pilot Wave Theories
(Herbert 49-50).
Outlook
Scientists continue to explore the
mysteries of quantum physics.
One recent experiment has detected the
formation of interference patterns
while the photons are still in flight
approaching the detector plane (Kocsis
1170). In another of series of
experiments, a group claims to have
seen increases in the sharpness of the
interference pattern of a twin-slit
setup when "trained meditators"
concentrated their attention on both
slits but reductions in interference
when the meditators were asked to look
away (Radin 157-71). It is
difficult to believe that merely
looking at the slits could enhance
quantum interference; identification
with "New Age" concepts is one of the
reasons that many professional
physicists regard inquiry into the
nature of wave function collapse as a
useless endeavor more appropriate to
metaphysics or philosophy than hard
science.
So which is
it—point-like particle or wave?
Probably both and neither. There
is no reason for the behavior of
microscopic objects to conform to our
conceptions. It may turn out to
be a matter of personal preference
whether one regards the particle
between the source and the screen as a
wave or as a point-like object being
pushed around by "quantum
forces". My main objection to
the Copenhagen concept is that it is
discontinuous. Copenhagenists
would have us believe that light
occupies the whole interference
pattern a pico-second before it hits
the screen, but becomes a point-like
object upon impact.
Do we live in a
deterministic universe or is God
really "playing with dice"? I think
that the jury is still out.
De-coherence theory provides a partial
answer to the paradox of Schrödinger's
Cat, but most experts do not see it as
the whole story. Given the
ability of the Copenhagen
interpretation to predict any results
observed by humans, one might ask, why
bother with other ideas? As one
theorist has observed, "The Copenhagen
Interpretation of quantum mechanics is
a remarkably efficient practical tool"
(D'Espagnat 258). I submit that
scientists should continue to search
for alternatives to the Copenhagen
interpretation. Valuable work
such as Bell's proof of quantum
non-locality and the likely role of
de-coherence are examples of why
Bohr's ideas should not be accepted as
the last word on the subject.
Notes
(1) During
World War II, Bohr was smuggled out of
Sweden to England in the bomb bay of a
Mosquito bomber. His oxygen mask
failed and Dr. Bohr lost
consciousness. Fortunately the
pilot saw the problem and reduced
altitude, saving Dr. Bohr's life.
(2) Some
two-photon sources provide photons
with exactly the same polarization;
some provide photons with exactly
opposite polarizations. The type
of source used does not alter the
validity of Bell's arguments.
(3) Photon polarization
is more complicated than suggested
herein, but the additional
complexities do not affect the
conclusions of the next paragraph.
(4) One point
that should be mentioned is that
quantum theory does not set a limit on
how large an object can be and still
be considered to have a wave
function. Presumably cats can be
represented by quantum wave functions,
whose state depends on interactions
with wave functions around them.
Experiments can be constructed such
that the state of a large object like
a cat depends on the collapse of the
wave function of a microscopic object
like a radioactive nucleus.
(5) Coherence is the
property of a quantum particle that
allows its corresponding wave to
interfere with itself.
De-coherence occurs when the wave
loses its well-defined wave character
through interactions with the
environment. Quantum theory
reduces to classical physics in the
limit of maximum de-coherence.
Works
Cited
D'Espagnat,
Bernard. Conceptual Foundations of
Quantum Mechanics. 2nd Ed.
Boston: Addison-Wesley, 1976. [This book
is intended for specialists.]
Ghirardi, Giancarlo. Sneaking a Look
at God's Cards: Unraveling the
Mysteries of Quantum Mechanics.
Princeton, NJ: Princeton University
Press, 2005.
Gribbin, John. Schrödinger's Kittens
and the Search for Reality: Solving
the Quantum Mysteries. NY: Little,
Brown, 1995.
Herbert, Nick. Quantum Reality:
Beyond the New Physics. NY: Anchor
Books, 1987.
Hermanns, Willliam. Einstein and the
Poet: In Search of the Cosmic Man.
Wellesley, MA: Branden Press, 1983.
Kaiser, David. How the Hippies Saved
Physics. NY: W.W. Norton, 2011.
Kocsis, S., et al. "Observing the
Average Trajectories of Single Photons
in a Two-Slit Interferometer." Science
332:3 (June 2011) 1170. [This paper
is intended for specialists.]
Nieuwenhuizen, Theo M. et al.
"Understanding quantum measurement from
the solution of a dynamical
model." Physics Reports
515:1 (April 2013), 1-166. [This
paper is intended for specialists.]
Penrose, Roger. The Emperor's New
Mind: Concerning Computers, Minds, and
the Laws of Physics. NY: Penguin
Books, 1989.
Radin, Dean, et al. "Consciousness and
the Double Slit Interference Pattern:
Six Experiments." Physics Essays
25:2 (2012), 157-171
Krebs
Biography
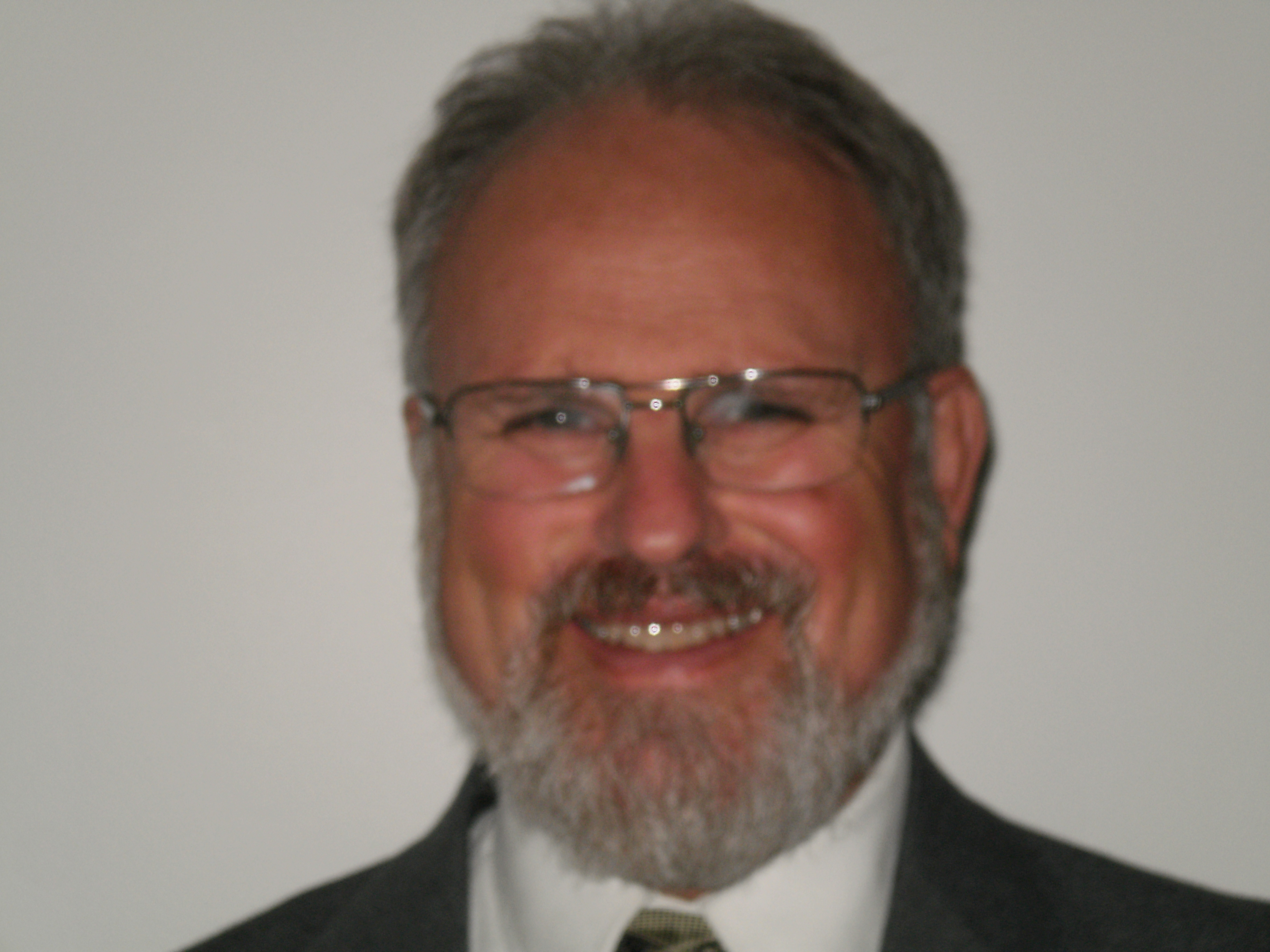
Danny Krebs is retired from the NASA
Goddard Space Flight Center in
Greenbelt, MD where he was a lead
engineer for space flight laser and
detector systems. Previously he was
at McDonnell Douglas Electronic
Systems Company in St. Louis, where he
did research leading to
all-solid-state high power lasers.
He has a B.S. in Engineering Physics
from the Colorado School of
Mines, M.S. degrees in
Engineering Management and Physics,
and a Ph.D. in Physics, all from
the University of Missouri-Rolla (now
Missouri University of Science and
Technology).
He has taught physics at Saginaw
Valley State University as both an
Adjunct Professor and full time
faculty. His paper titled
"Personal Transportation in the 21st
Century and Beyond" won the 2011
Paxton Award.
"Saving Dr. Schrödinger's Cat" was
presented to the Saginaw Valley Torch
Club on January 7, 2014.